By Jim Gawel, Ph.D., Anthony Endresen, Erin Hull, Amina Kedir, and Corey King, Environmental Science and Studies, School of Interdisciplinary Arts and Sciences, University of Washington Tacoma, Rebecca Neumann, Ph.D., and Pam Barrett, Ph.D., Civil and Environmental Engineering, University of Washington.
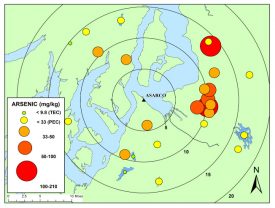
Figure 1: Arsenic concentrations in surface sediments in Puget Sound Lowland lakes (Gawel et al. 2014).
Arsenic, a priority Superfund contaminant, neurotoxin and carcinogen, is a ubiquitous contaminant polluting many urban surface water bodies. (A majority of Superfund sites are located in densely settled areas and 139 sites have arsenic-contaminated surface waters.) However, the human health and ecological implications of this contamination are unclear due to an incomplete understanding of arsenic bioavailability in urban waters, which are typically affected by eutrophication.
Current surface water quality criteria set by the EPA recommend that, in freshwater, total arsenic concentrations be below 150 µg/L when aquatic organisms are chronically exposed to arsenic and below 0.14 µg/L when the aquatic organisms are consumed by humans (the human health guideline is under revision). Bioaccumulation and ecological toxicity of arsenic appear to be linearly related to water concentrations in laboratory experiments, but these relationships are often not transferable to natural environments. In complex natural environments, total aqueous arsenic concentration is not the best indicator of bioaccumulation and ecological toxicity, so may not be the proper benchmark for protecting human and environmental health. The challenge is to understand what factors control the bioavailability of arsenic. The literature suggests that arsenic speciation, sediment concentrations, and the presence of other solutes, such as phosphate and dissolved organic matter, are potentially important variables.
The Puget Sound Lowland region contains numerous urbanized lakes downwind of the former ASARCO (American Smelting and Refining Company) copper smelter in Tacoma now designated the Commencement Bay/Nearshore Tideflats Superfund Site. Sediments in these lakes accumulated arsenic emitted by the smelter, and those sediments now represent a long-term source of contamination to surface water. Our initial mapping of arsenic contamination in lake sediments and water column arsenic contamination shows the extent of contamination in area lakes [Figure 1, Gawel et al. 2014].
The region includes lakes with arsenic-contaminated waters that display different redox behaviors, ranging from seasonally stratified and anoxic to well-mixed and oxygenated. Redox refers to a transfer of oxygen molecules, in which some elements are gaining oxygen (oxidizing) and others are losing it (reduction).
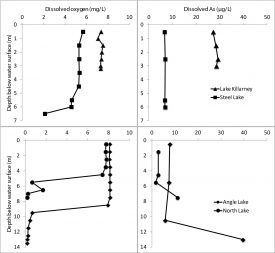
Figure 2: Dissolved oxygen and arsenic profiles in the four study lakes showing the difference between stratified and well-mixed lakes.
Our four current study lakes are all in King County, Washington, downwind of the smelter: Angle Lake (>200 ppm As in sediments) and North Lake (85 ppm As) are deeper, summer-stratified lakes, and Lake Killarney (>200 ppm As) and Steel Lake (48 ppm As) are shallow, well-mixed lakes. Elevated levels of arsenic usually appear in anoxic bottom waters that develop when lakes thermally stratify during the summer; anoxic conditions promote the dissolving of arsenic-bearing minerals in sediments. However, we have found that the Puget Sound Lowland region also contains lakes that maintain elevated aqueous arsenic concentrations under oxic conditions [Figure 2].
The mechanisms responsible for an oxic, arsenic-contaminated water column are unclear — arsenic usually partitions to the solid phase in oxic conditions and would be expected to settle to the lakebed. The situation in Puget Sound Lowland lakes raises questions about the physical and geochemical processes controlling arsenic concentrations in oxic waters, and highlights the potential for enhanced aquatic exposure to arsenic. As plankton and fish cannot survive long in anoxic waters, lake redox status (controlled by the presence or absence of dissolved oxygen) greatly impacts the spatial overlap between arsenic contaminants in the water and suitable biological habitat. Lake redox status is a master variable, regulating chemical speciation, major ion chemistry, and organism behavior, and yet is not formally considered when evaluating arsenic toxicity in aquatic systems.

Figure 3: Average arsenic concentrations in different aquatic macrophyte types in lakes in the Puget Sound Lowlands; error bars are large due to variability in arsenic levels and species found in lakes sampled.
Some of our preliminary results on arsenic transfer into plants and animals include accumulation in aquatic macrophytes, benthic macroinvertebrates, phytoplankton and zooplankton. In aquatic macrophytes, undergraduate Anthony Endresen’s initial work shows that arsenic is at much higher concentrations in submergent plants than in floating or emergent plants [Figure 3]. Anthony will work this summer to expand his sampling and correlate with arsenic concentrations in the water column.
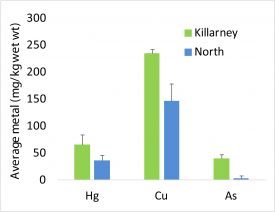
Figure 4: Average mercury, copper and arsenic concentrations in Chironomid larvae (bloodworms) in two arsenic contaminated lakes.
In her research, undergraduate Amina Kedir has found that higher sediment concentrations do result in greater arsenic uptake in benthic macroinvertebrates [Figure 4]. She is currently analyzing samples from additional lakes and this summer will look at arsenic levels in emerging adults.
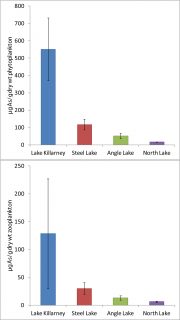
Figure 5: Average arsenic concentrations in (a) phytoplankton and (b) zooplankton collected July-September 2015 from four study lakes showing effect of overlap between oxygenated waters and arsenic uptake in Lake Killarney and Steel Lake.
Finally, undergraduate Erin Hull and collaborators Corey King, Pam Bartlett and Rebecca Neumann have found strong evidence of the impact of overlapping oxygenated waters and dissolved arsenic [Bartlett et al. manuscript submitted]. Their results show a strong effect of this phenomenon on arsenic accumulation in plankton [Figure 5]. Moreover, taken together, these results also show potential for arsenic accumulation in fish as they eat both benthic macroinvertebrates and zooplankton.
Because these lakes sustain a variety of freshwater biota and are routinely fished by a diverse urban population, it is critical to understand not only how arsenic-contaminated lake waters develop, but the effect they have on aquatic life and the risk they pose to human health. This study will inform management strategies to minimize the negative effects of arsenic in urban aquatic environments.
Sources:
Gawel, J.E., J.A. Asplund, S. Burdick, M. Miller, S.M. Peterson, A. Tollefson, and K. Ziegler (2014) Arsenic and lead distribution and mobility in lake sediments in the south-central Puget Sound watershed: The long-term impact of a metal smelter in Ruston, Washington, USA. Sci. Total Environ., 472: 530-537.